Plumes.
Plumes permeate all coronal holes, yet are invisible in the solar wind.
How this variable, filamented flow becomes the uniform fast wind in unknown.
Whether this is related to the source and evolution of MHD turbulence in
the solar wind will be answered by Solar Probe.
The picture on the right is a composite of SOHO/EIT, HAO Mk3 coronagraph,
and SOHO/LASCO C2 showing plumes in the northern polar coronal hole in
March 1996 (DeForest et al.). The plumes are seen to originate in the photosphere,
diverge along with the coronal hole boundaries, and extend here to at least
6 Rs. Recent (1999) observations show plumes are sometimes detectable
out to 15-30 Rs.
A plume will tend to last for several days but within a plume there
are brightness fluctuations on much shorter time scales.
Solar Probe will fly directly through plumes in the polar coronal holes. |
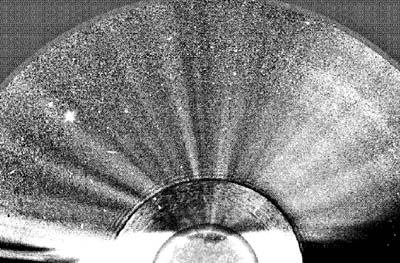 |